This article delves into the detailed workings of multijunction solar cells, their structure, advantages over conventional solar cells, and their potential impact on the future of renewable energy.
Multijunction solar cells represent a significant leap in solar technology, enhancing energy conversion efficiency to 40% as compared to conventional single junction solar cells (20% average). Their ability to capture a broader range of the solar spectrum makes them a promising solution for high-efficiency power generation, particularly in applications like space exploration and concentrated photovoltaic systems (CPVs).
1. Understanding Conventional Solar Cells:
Before diving into multijunction solar cells, it’s essential to understand the basics of conventional solar cells.
How Conventional Single Junction Solar Cells Work?
Conventional solar cells, typically made from silicon, operate on the photovoltaic effect. When sunlight strikes the silicon wafer, it excites electrons, creating electron-hole pairs. These electrons are then directed to an electrical circuit, generating electricity. The key factor influencing their efficiency is how much of the solar spectrum can be absorbed and converted into usable electricity.
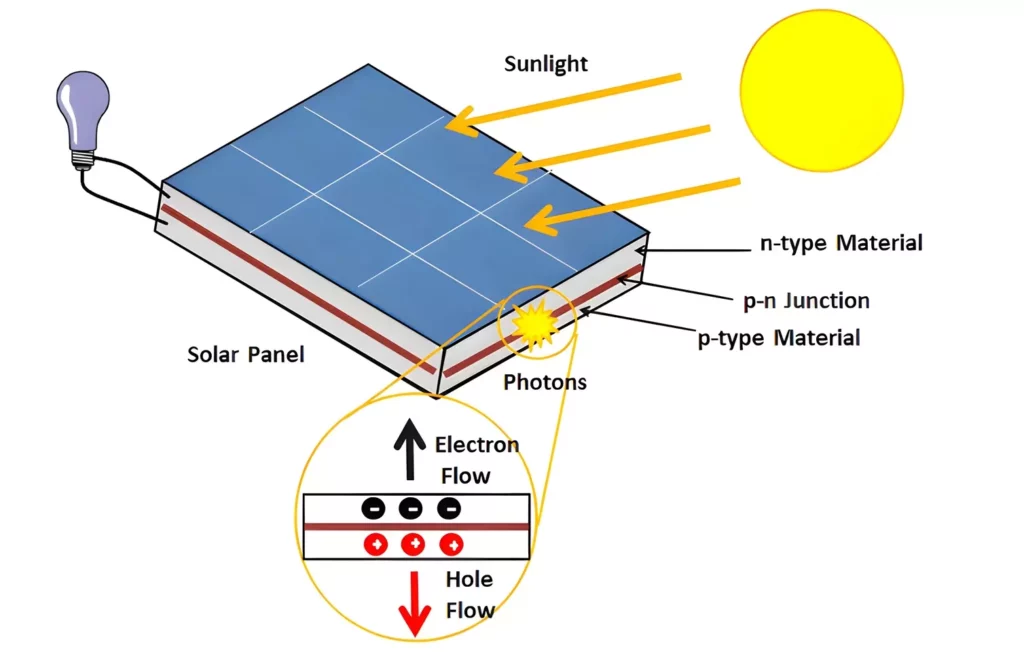
Types of Conventional Solar Cells:
- Monocrystalline Silicon Cells (Mono-Si): These are made from a single crystal structure, providing higher efficiency (up to 22-24%) due to better electron flow.
- Polycrystalline Silicon Cells (Poly-Si): These are less expensive to produce but are slightly less efficient (15-20%) due to grain boundaries that scatter electrons.
- Thin-Film Solar Cells: These are lightweight and flexible but generally offer lower efficiency (10-12%).
Efficiency Limitations
Conventional solar cells are limited by the Shockley-Queisser limit, which dictates the maximum theoretical efficiency of a single-junction solar cell at around 33%. This limit arises because a single material can only absorb a specific portion of the solar spectrum. The majority of sunlight’s energy, particularly photons with higher or lower energies, either passes through the cell or is converted to heat rather than electricity.
2. Introduction to Multi-Junction Solar Cells:
Multijunction solar cells (MJSCs) aim to surpass the efficiency limits of conventional cells by layering multiple semiconductor materials, each designed to absorb a different portion of the solar spectrum. By doing this, multijunction cells can capture more energy from sunlight, drastically improving their efficiency.
Structure and Working Principle
Multijunction solar cells consist of multiple layers or “junctions,” each with different bandgaps (the energy required to excite electrons). The topmost layer has the highest bandgap and absorbs high-energy photons (such as ultraviolet light). Photons with lower energy pass through to the lower layers, where they are absorbed by materials with smaller bandgaps (optimized for visible and infrared light).
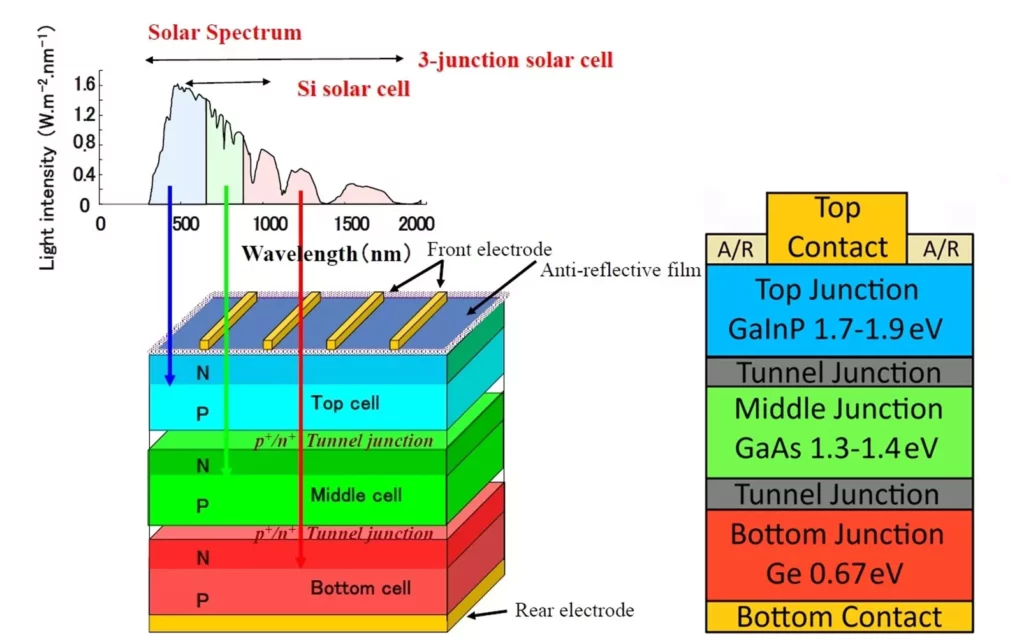
- The top cell might be made of Gallium Indium Phosphide (GaInP), which captures blue and ultraviolet light.
- The middle cell could be composed of Gallium Arsenide (GaAs), which absorbs green light.
- The bottom cell is typically Germanium (Ge), designed to absorb infrared light.
Each junction operates like an individual solar cell, and the combination of these layers ensures that more photons from different parts of the solar spectrum are captured, which increases the overall energy conversion efficiency.
Key Materials Used in Multi-Junction Cells:
- III-V Semiconductors: Gallium arsenide (GaAs), gallium indium phosphide (GaInP), and germanium (Ge) are widely used due to their optimal bandgap properties.
- Perovskites: A newer class of materials being investigated for multijunction cells due to their tunable bandgaps and high efficiency.
The choice of materials depends on the application, whether it is terrestrial or extraterrestrial.
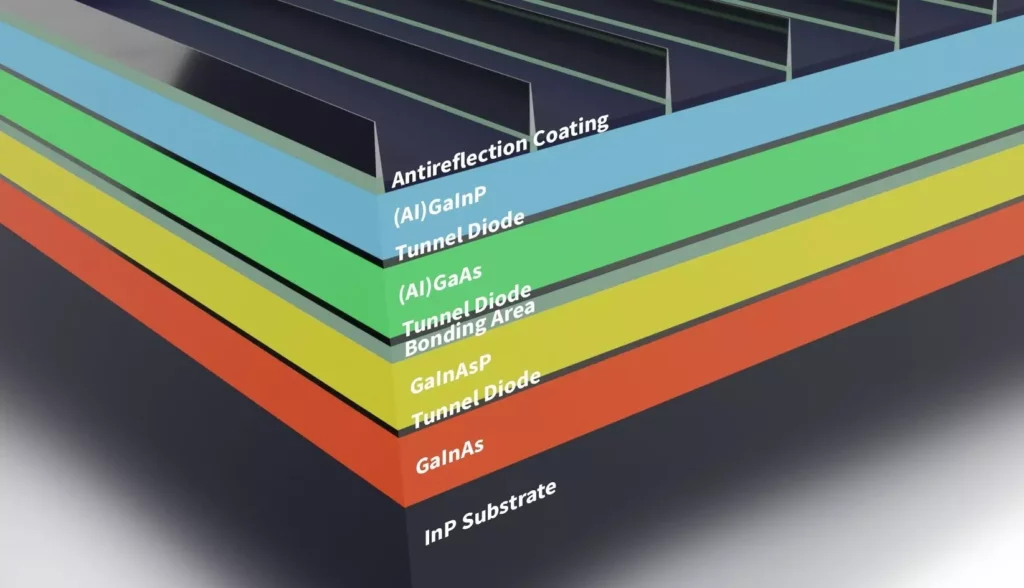
3. Manufacturing of Multi-Junction Solar Cells:
Here is a general overview of the manufacturing process of multi-junction solar cells:
1. Material Selection
- Semiconductor Materials: Multi-junction solar cells typically use materials from the III-V semiconductor family, such as Gallium Arsenide (GaAs), Indium Phosphide (InP), Indium Gallium Phosphide (InGaP), or Gallium Indium Arsenide (GaInAs).
- These materials are selected because they can be tailored to absorb specific portions of the solar spectrum, typically using bandgap engineering.
2. Epitaxial Growth (MOCVD or MBE)
- Epitaxial Growth is the process used to grow the multi-layer structure of the solar cell. Two main techniques are employed:
- Metal-Organic Chemical Vapor Deposition (MOCVD): This is the most common method, where metal-organic gases (e.g., trimethylgallium) and hydrides (e.g., arsine) are introduced into a reactor to deposit thin, high-quality layers of semiconductor material on a substrate.
- Molecular Beam Epitaxy (MBE): In this technique, pure elements are heated in a vacuum to form atomic beams, which are directed onto the substrate to form crystalline layers. MBE offers precise control over layer thickness but is more costly and slower than MOCVD.
- The different junctions (or layers) are stacked on top of each other:
- Top junction: Absorbs high-energy (short-wavelength) light.
- Middle junction(s): Absorbs medium-energy light.
- Bottom junction: Absorbs low-energy (long-wavelength) light.
3. Substrate Preparation
- Substrate selection is critical. Common substrates include Germanium (Ge) or Gallium Arsenide (GaAs), which serve as a base for the epitaxial growth.
- Before deposition, the substrate is carefully cleaned to remove impurities and ensure proper layer growth.
4. Tunnel Junctions
- Between each semiconductor layer, tunnel junctions are added. These are ultra-thin layers of highly doped material that allow electrical current to flow between the stacked junctions while minimizing electrical losses.
- The tunnel junctions facilitate the movement of charge carriers between the different bandgap materials.
5. Anti-Reflection Coatings
- After the epitaxial growth and assembly of the layers, the surface of the multi-junction cell is coated with an anti-reflection layer. This coating, typically made from silicon nitride (Si₃N₄), minimizes light reflection off the surface, ensuring that as much light as possible is absorbed into the cell.
6. Metal Contact Formation
- Metallic contacts are deposited onto the top and bottom surfaces of the solar cell to collect the generated electric current. The top contact is usually a grid or mesh structure to minimize light blockage, while the bottom contact is often a solid layer.
- Metals like silver (Ag) or aluminum (Al) are commonly used for their high conductivity.
7. Cell Isolation and Dicing
- Once the entire solar cell structure is grown, the wafer is diced into individual solar cells using methods like laser scribing or diamond saw cutting.
- Edge isolation may be performed to electrically isolate the cells from each other and prevent short circuits.
8. Encapsulation and Packaging
- For protection and durability, the solar cells are often encapsulated in a protective material, such as glass or polymer layers. Encapsulation shields the cells from environmental damage, such as moisture and mechanical stress.
- Cells are typically assembled into modules for commercial applications, often using a thin plastic film for space applications or more robust packaging for terrestrial applications.
9. Testing and Quality Control
- Electrical Testing: Each solar cell undergoes rigorous testing to measure performance characteristics like efficiency, current, voltage, and fill factor.
- Spectral Testing: Multi-junction cells are tested under standard solar conditions, typically using AM1.5 spectrum simulators, to ensure they perform well across various wavelengths.
- Thermal and Environmental Stress Testing: The cells are also tested for durability under extreme environmental conditions (temperature, humidity, etc.).
4. Advantages of Multi-Jnction Solar Cells:
Higher Efficiency
The most significant advantage of multijunction solar cells is their high efficiency. While single-junction silicon cells have a theoretical maximum efficiency of 33%, multijunction cells can achieve efficiencies exceeding 40% in laboratory settings, with some even reaching 50% under concentrated sunlight. This is because each layer in a multijunction cell is designed to harvest different wavelengths of light, maximizing energy capture.
Better Performance in Harsh Conditions
Multijunction solar cells are especially useful in space applications, where their superior efficiency under high-intensity and varied light conditions is a significant advantage. They are more resistant to damage from high-energy particles, making them ideal for satellites and space missions.
Concentrated Photovoltaic Systems (CPVs)
Multijunction cells are often used in concentrated photovoltaic systems, where sunlight is focused onto the cell using lenses or mirrors. Since MJSCs can handle higher light intensities and convert more of that light into electricity, they are well-suited for CPVs. In these systems, fewer cells are needed, reducing material costs despite the higher cost per cell.
Flexible Design
By adjusting the bandgaps of the layers, engineers can design multijunction cells tailored to specific applications, optimizing performance based on location, atmospheric conditions, and the solar spectrum.
5. Disadvantages of Multi-Junction Solar Cells:
Complexity and Cost
One of the major challenges with multijunction cells is their complexity. Layering different semiconductor materials requires sophisticated manufacturing processes. This makes MJSCs significantly more expensive to produce than conventional silicon cells. The use of rare and expensive materials, like gallium and indium, further increases the cost.
Manufacturing Difficulties
The layers in multijunction solar cells need to be carefully grown with minimal defects to ensure high performance. This requires high-precision manufacturing techniques such as molecular beam epitaxy (MBE) or metal-organic chemical vapor deposition (MOCVD). These processes add to the production time and cost, limiting the mass-market potential of MJSCs.
Thermal Management
With multijunction solar cells, particularly in CPV systems, heat management is crucial. Since these cells can operate under concentrated sunlight, managing the heat produced is necessary to prevent damage and ensure long-term reliability.
6. Single Junction Solar Cells vs Multi Junction Solar Cells:
Feature | Single Junction Solar Cells | Multijunction Solar Cells |
---|---|---|
Efficiency | 15-22% (commercial), up to 27% (lab) | 30-40% (commercial), 50%+ (lab, under CPV) |
Materials | Primarily silicon | III-V compounds (GaAs, GaInP, Ge), Perovskites |
Cost | Relatively low | Expensive due to complex materials and processes |
Manufacturing | Simple and widely available processes | Complex and precision-dependent |
Optimal Use | Rooftop installations, utility-scale | Space applications, CPV, high-efficiency demands |
Response to Solar Spectrum | Limited by single bandgap | Multiple layers harvest a broader spectrum |
Durability | Good in general terrestrial conditions | Superior in space or harsh environments |
7. Applications of Multi-Junction Solar Cells:
Multijunction solar cells find their most significant applications in specialized fields, where their high efficiency justifies the cost.
Space Exploration
The power needs of satellites, space stations, and exploratory missions have led to widespread adoption of MJSCs in the aerospace industry. Their high efficiency, durability, and ability to perform under extreme conditions make them the go-to technology for solar power in space.
Concentrated Photovoltaics (CPV)
On Earth, multijunction solar cells are used in CPV systems to generate electricity more efficiently by focusing sunlight onto smaller, more expensive cells. CPV systems are especially viable in regions with high direct sunlight, such as deserts.
High-Efficiency Solar Panels
Though currently too expensive for widespread residential use, multijunction solar panel may eventually find their way into high-end commercial or utility-scale solar farms as manufacturing processes improve and costs decrease.
8. The Future of Multi-Junction Solar Cells:
While conventional silicon-based solar cells will likely remain the dominant technology for residential and commercial applications due to their low cost, multijunction solar cells represent the future of high-efficiency solar technology. Advances in materials science, particularly the development of perovskite-based multijunction cells, offer the potential for cheaper production and improved performance, further closing the gap between cost and efficiency.
In addition, ongoing research into flexible and lightweight MJSCs may open the door to new applications, such as integrating solar cells into textiles or portable devices, expanding the reach of solar energy beyond traditional infrastructure.
Conclusion:
Multijunction solar cells present a powerful solution for overcoming the efficiency limitations of conventional solar technology. By capturing more of the solar spectrum, they offer the highest efficiencies achievable with today’s technology. Although their cost and complexity currently limit widespread use, their applications in space, CPV, and potentially high-end solar installations showcase their potential to revolutionize the solar industry. As research progresses and manufacturing techniques improve, multijunction cells could become an essential part of the global transition to renewable energy.